Introduction 介紹
本文討論了供應(yīng)鏈的考慮和使用生物質(zhì)燃料的發(fā)電站在一個(gè)大的規(guī)模上的成本和成本。這是在這個(gè)規(guī)模的物流使用生物質(zhì)燃料供應(yīng)可能是復(fù)雜的和潛在的問(wèn)題,物流成本將有一個(gè)重要的軸承總交付成本的生物量(即總累積成本的生物質(zhì)燃料在交付給一個(gè)發(fā)電站)。它是要認(rèn)識(shí)到,物流成本與物流活動(dòng)的一體化管理,可以對(duì)一個(gè)產(chǎn)品或產(chǎn)業(yè)的成敗至關(guān)重要,尤其在一個(gè)新興行業(yè)的案例(巴羅,1992)。本文的工作是根據(jù)威斯敏斯特大學(xué)和蘇格蘭農(nóng)業(yè)學(xué)院(艾倫等,1996)進(jìn)行的一個(gè)研究項(xiàng)目。它集中在供應(yīng)鏈組件從收獲的點(diǎn),通過(guò)交付在發(fā)電站。這項(xiàng)研究評(píng)估了潛在的供應(yīng)系統(tǒng)的燃料供應(yīng)站,計(jì)算出這些供應(yīng)鏈的交付成本,并確定了各種系統(tǒng)的相對(duì)優(yōu)勢(shì)和生物質(zhì)燃料供應(yīng)的環(huán)境影響。本文首先討論的原因,在使用生物質(zhì)燃料作為能源的增加,在生物質(zhì)燃料供應(yīng)的活動(dòng)和物流規(guī)劃和管理的重要性,提供生物質(zhì)燃料發(fā)電廠的興趣增加。然后,它集中在四種生物質(zhì)燃料的供應(yīng)鏈的不同的供應(yīng)鏈模型所使用的方法。每一個(gè)生物質(zhì)燃料的結(jié)果進(jìn)行了總結(jié),然后提出了一個(gè)比較,交付的所有這些燃料的成本[ 1 ]。本文的結(jié)論,討論適當(dāng)?shù)囊?guī)劃,管理和操作方法,生物質(zhì)燃料供應(yīng)的研究建議。作者要感謝貿(mào)易和工業(yè)部資助項(xiàng)目,本文是基于對(duì)管理這個(gè)項(xiàng)目法。This paper addresses the supply chain considerations and costs of using biomass fuel on a large scale for electricity generation at power stations. It is at this scale of use that the logistics of biomass fuel supply are likely to be both complex and potentially problematic, and logistics costs will have an important bearing on the total delivered cost of biomass (i.e. the total cumulative cost of biomass fuel at the point of delivery to a power station). It is important to recognise that logistics costs and the integrated management of logistics activities can be vital to the success or failure of a product or industry, especially in the case of a fledgling industry (Ballou, 1992). The work in this paper is based on a research project conducted by the University of Westminster and the Scottish Agricultural College (Allen et al., 1996). The aim of the project was to examine the options for supplying the end user with biomass fuel of the right specification, in the right quantity at the right time from resources which are typically diverse and often seasonally dependent. It concentrated on the supply chain components from the point of harvesting through to delivery at the power station. The study assessed potential supply systems for the supply of fuel to power stations, calculated the delivered costs of these supply chains, and identified the relative advantages of the various systems and the environmental impacts of biomass fuel supply. The paper first addresses the reasons for the increase in interest in the use of biomass fuel as an energy source, the activities involved in biomass fuel supply and the importance of logistics planning and management in supplying biomass fuel to power stations. It then concentrates on the approach used to model the delivered costs of different supply chains for four biomass fuels. A summary of the results for each of the biomass fuels is then presented, with a comparison of delivered costs for all of these fuels[1]. The paper concludes by discussing appropriate planning, management and operational approaches to biomass fuel supply suggested by the research. The authors wish to thank the Department of Trade and Industry for funding the project on which this paper is based and ETSU for managing this project. #p#分頁(yè)標(biāo)題#e#
Biomass fuel and its use for electricity generation 生物質(zhì)燃料及其用于發(fā)電的應(yīng)用
Biomass is the living material produced on the planet by the process of photosynthesis. Biomass has been used for thousands of years as a source of energy and is today a major fuel in terms of the quantities used world-wide. In fact, more people depend on biomass for energy world-wide than on any other fuel (UN, 1991). Much of this biomass use is in very small- scale applications including domestic cooking and heating. By contrast, biomass is still relatively little used in electricity generation in Europe, not only in comparison to fossil fuels and nuclear power, but also to other renewable sources including hydro, wind and energy from waste. A number of different primary fuels are often referred to as biomass and these are often categorised in four groups: (1) wood (such as forest fuel available from the felling of young trees and thinning operations or from trees such as willow and poplar grown on a short rotation basis specifically as a biomass fuel); (2) crop residues such as cereal straw; (3) energy crops grown specifically for biomass such as high energy yielding grasses including miscanthus, spartina and reed canary grass; (4) human and animal excrement including cow, pig and poultry slurry. There are a range of conversion technologies available for making use of biomass. The primary fuels can either be used directly (for example, burning wood on a stove to produce heat) or can be converted into secondary fuels such as liquid or gaseous fuels through the use of technologies such as gasification, pyrolysis and anaerobic digestion. Both primary and secondary biomass fuels can be used to produce heat, mechanical power or electricity (or a combination of these). In the developed world, biomass fuels are often used to produce both district heating and electricity. In Scandinavia, district heating schemes providing heat and hot water for numerous towns have existed for several decades. In addition, some of these plants have the capability to produce combined heat and power thereby also providing electricity. In the UK, biomass has not been largely used as a source of energy in recent decades. However, the UK government is currently making efforts and providing funding arrangements with the intention of establishing electricity-generating facilities fuelled by biomass fuel (Deprtment of Trade and Industry, 1994). There is a significant existing biomass resource base in the UK and this could expand rapidly if agricultural land, taken out of food production as a result of set-aside arrangements, is turned over to growing biomass (ETSU, 1994). Research and demonstration schemes in which biomass fuels are used to generate electricity already exist in the UK, however, most of these pilot schemes are of an extremely small scale and use biomass produced on the farm or in the forest in which they are located. However, within the next few years #p#分頁(yè)標(biāo)題#e#
many more larger scale power stations are likely to be developed. While the energy generated by these plants will initially be subsidised by government it is intended that they would be producing electricity at commercially competitive prices within 15 years (Department of Trade and Industry, 1994). At present, however, producing electricity from biomass is far more expensive than from other more traditional means such as coal and gas, partly as a result of the logistics costs involved in fuel supply. Therefore a significant amount of research and learning by experience will be necessary if biomass is to prove competitive with fossil fuels in the longer term. One of the main objectives of the project involved developing suitable supply strategies from the point of production to point of utilisation for different types of biomass fuel such as straw, forest fuel, short rotation coppice, high-energy yielding grasses and animal waste that are both efficient and economic. While there is a need for such supply systems to be economically efficient in order to compete with other sources of electricity it is also necessary that the environmental impacts of the proposed biomass supply chains are minimised. This is very important as the rationale for using biomass fuel is that it is less harmful to the environment than traditional fossil fuels for the following reasons: . it is a more sustainable form of fuel than fossil fuel; . the increased use of biomass fuel, together with reductions in the use of fossil fuel, will help to reduce emissions of pollutant gases which contribute to acid rain and greenhouse gas levels; . the role that forestry and coppice can play in encouraging wildlife. However, it should be recognised that the production and use of biomass fuels also result in some negative environmental impacts. These include: . that the introduction of novel agricultural crops on a large scale could have serious ecological consequences in its own rights, and may be little better than other intensive agricultural crops in terms of wildlife habitat; and . that many analysts view set aside land not as a waste of productive space but as an important opportunity to mitigate the ecological damage caused by intensive agriculture, which would be foregone in the event of a straight substitution of non-food crops.
The biomass fuel supply chain 生物質(zhì)燃料供應(yīng)鏈
The biomass supply chain is made up of a range of different activities. These can include ground preparation and planting, cultivation, harvesting, handling, storage, in-field/forest transport, road transport and utilisation of the fuel at the power station. Given the typical locations for biomass fuel sources (i.e. on farms and in forests) the transport infrastructure is usually such that road transport will be the only potential mode for collection of the fuel. Other factors that favour the use of road transport include the distances over which the fuel is transported (which tend to be relatively short) and the greater flexibility that road transport Costs of biomass fuel supply #p#分頁(yè)標(biāo)題#e#
IJPDLM 28,6 can offer in comparison with other modes. In order to supply biomass from its point of production to a power station the following activities are necessary: . Harvesting of the biomass in the field/forest. . In-field/forest handling and transport to move the biomass to a point where road transport vehicles can be used. . Storage of the biomass. Many types of biomass will be harvested at a specific time of year but will be required at the power station on a year-round basis, it will therefore be necessary to store them. The storage point can be located on the farm/forest, at the power station or at an intermediate site. . Loading and unloading road transport vehicles. Once the biomass has been moved to the roadside it will need to be transferred to road transport vehicles for conveyance to the power station. At the power station the biomass will need to be unloaded from the vehicles. . Transport by road transport vehicle. Using heavy goods vehicles (rather than agricultural or forestry equipment) for transport to the power station is likely to be essential due to the average distance from farms to power station, and the carrying capacity and road speed of such vehicles. . Processing of the biomass to improve its handling efficiency and the quantity that can be transported. This can involve increasing the bulk density of the biomass (e.g. processing forest fuel or coppice stems into wood chips) or unitising the biomass (e.g. processing straw or miscanthus in the swath into bales). Processing can occur at any stage in the supply chain but will often precede road transport and is generally cheapest when integrated with the harvesting.
Management and planning of the biomass fuel supply chain 生物質(zhì)燃料供應(yīng)鏈管理與規(guī)劃
The activities that take place in biomass fuel supply chains are highly interconnected. While this point is often referred to in logistics and supply chain literature, it is often difficult to appreciate (for instance, see Ballou, 1992; Christopher, 1987; Cooper, 1993). The biomass supply chain is very transparent and it is easy to understand how upstream decision-making affects later activities in the chain. For instance, trees grown on farmland on a short rotation coppice basis can either be harvested as five metre whole sticks or cut and immediately processed into wood chips. Different harvesting machinery is required for each of these harvesting systems. However, this is only the beginning of differences in the supply chain. While sticks can be stored on the edge of fields without experiencing decomposition, chips need to be stored on at least a hard standing, and in a covered environment if decomposition is to be prevented. Therefore, the storage requirements for the two products are very different. Similarly, sticks and chips require very different transport systems in terms of both handling (i.e. loading and unloading vehicles) and suitable transport vehicle bodies for carrying them (see Figure 1). #p#分頁(yè)標(biāo)題#e#
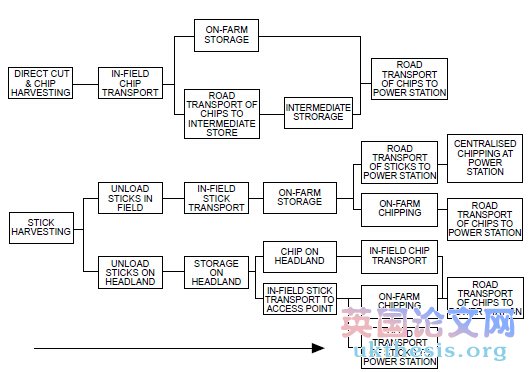
ON-FARM STORAGE ROAD TRANSPORT OF CHIPS TO INTERMEDIATE ROAD TRANSPORT OF CHIPS TO POWER STATION DIRECT CUT IN-FIELD & CHIP CHIP HARVESTING TRANSPORT STRORAGE CENTRALISEDROAD INTERMEDIATE STORE CHIPPING AT TRANSPORT POWER HEADLAND IN-FIELD STICKTRANSPORT TO TRANSPORT ON-FARM OF STICKS TO STATION POWER STATION UNLOAD IN-FIELD ON-FARM STICKS IN STICK STORAGE ON-FARM ROADFIELD TRANSPORT CHIPPING TRANSPORT OF CHIPS TO POWER STATION CHIP ON IN-FIELD CHIP UNLOAD STORAGE STICKS ON ON HEADLAND HEADLAND ROAD TRANSPORT CHIPPING OF CHIPS TO ACCESS POINT POWER STATION STICK HARVESTING ROAD TRANSPORT OF STICKS TO POWER STATION From this one simple example it is possible to understand the degree of interconnection between the different activities and thereby appreciate the need to take a total supply chain perspective when planning any single activity in the chain rather than considering that activity in isolation. The easiest and most cost-effective harvesting system can result in the need for expensive storage systems and can even lead to an inability to supply fuel of the desired quality to the power station. Therefore the harvesting approach fundamentally affects the storage, handling and transport requirements in the supply chain. Similarly, if planning the supply system from the other end of the chain as is common, the choice of power station technology, size and location will dictate how all the upstream activities are to be conducted so that biomass arrives at the power station at the correct time, in the correct quantity, and in the desired shape, size and quality. This interconnectedness is also true of environmental impacts in the biomass fuel supply chain. For instance, as previously discussed, the harvesting system will determine the type of storage and transport system that has to be used. Even if an alternative approach to storage and transport is known to be less harmful to the environment, the chosen harvesting system can preclude its selection. For example, there are concerns about the possible health implications for workers and the public of spores produced by coppice wood chips stored in piles. Storage of the coppice as whole sticks would reduce these risks. However if the coppice has been chipped at the point of harvest, storage in stick form is not possible. The environmental impact of biomass fuel supply is of great importance as the rationale driving the use of biomass fuel is that it is less harmful to the environment than traditional fossil fuels. The activities that occur in the biomass Costs of biomass fuel supply Figure 1. Supply chain options for shore rotation coppice
IJPDLM 28,6 supply chain can be responsible for a number of environmental impacts; these can include noise, fossil fuel use and emissions, visual intrusion, health and safety issues, water pollution and traffic generation. It is of fundamental importance that the environmental benefits and energy production that results from using biomass fuel outweighs the environmental impacts and resource consumption that their growth and supply incur. The most logistically efficient supply chain or the one producing the lowest delivered costs will not necessarily provide the best benefit to cost ratio in environmental terms. The biomass supply chain is made up of a range of different parties including farmers, forest owners, agricultural and forestry contractors, transport and distribution companies, fuel suppliers (i.e. organisations taking the risk and responsibility for supplying fuel to power stations), and power station operators. Given the large number of different organisations involved it is essential that they work closely together in planning and co-ordinating fuel supply if the correct quantity and specification of biomass is to arrive in a timely manner at the power station. These supply chain parties differ in a number of respects including their outlooks, priorities and scale of operation. These complications make it even more necessary to take a supply chain view. #p#分頁(yè)標(biāo)題#e#
Logistics requirements in biomass supply 生物質(zhì)供應(yīng)物流需求
Biomass power stations are small in terms of generating capacity in comparison with fossil fuel powered stations; biomass stations will typically be 5-20 megawatts (MW) compared with, say, 2,000MW for a coal power station. However, they require significant quantities of biomass fuel; in the case of straw, for example, a 20MW plant will require approximately 150,000 tonnes of straw annually. This is explained by the low calorific value of biomass compared to coal or oil. The low bulk density of most biomass fuels means that the volume occupied by a given tonnage will far exceed that occupied by the same tonnage of fossil fuel, thereby resulting in the need for a much larger number of lorry movements. This creates logistical problems in terms of the volume of biomass that has to be handled, stored and transported. Table I shows the annual biomass requirement and hence the quantity of fuel that has to be handled and the number of road transport vehicle deliveries that would typically be necessary at a 20MW straw-fired power station and a 10MW coppice station. Type and size Annual biomass Road transport vehicle of power station requirement (wet tonnes) deliveries per daya 20MW straw-fired 150,000 40 10MW coppice-fired 135,000 24 Table I. Note: aTypical fuel requirements assumes the use of a 35 tonnes gross weight flatbed lorry for straw bale transport and a 38 for biomass power station tonne gross weight tipping lorry for coppice chip transport
The relatively low economic and calorific values and low bulk densities of biomass fuels result in the transport and logistics arrangements (including storage, handling, loading and unloading) involved in biomass supply from farms and forests to power stations being responsible for a significant proportion of total delivered fuel cost. It is therefore of great importance that biomass fuel supply is conducted in an efficient manner so as to control the logistics costs, and hence delivered costs of the fuel. Biomass transport Transport is an important element in the supply of biomass fuel to the power station, linking together all the activities that have to take place between the point of production through to the point of use at the power station and the locations at which they occur. As already explained, road transport is likely to be the chosen mode for moving biomass from farms/forests to power stations (due to the typically rural location of the biomass fuel and the relatively short distances over which the fuel is moved). The catchment area for the biomass resource and hence the transport distance over which biomass will have to be moved between point of production, storage and power station will depend on a number of factors. These include the size of the power station and the conversion technology used, and hence the quantity of biomass fuel required; the crop yield that is achieved; the planting density around the power station and the availability of the material for biomass resource (e.g. straw has competing uses and therefore only a proportion of the total produced will be available for use in biomass schemes). Biomass storage Most electricity generating stations to which the biomass fuels are supplied will have very limited on-site storage facilities. This is due to the space and facilities required to hold stock and the physical and financial costs of holding stock. In the case of biomass that is harvested over a relatively short period of the year, such as straw and short rotation coppice, large quantities will have to be stored in order that the supply of fuel is spread evenly over the course of a year. This will require storage facilities either where the fuel is produced or at intermediate points. The size of the storage facility at the power station will also affect the transport arrangements. A power station with a relatively small on-site stock level (e.g. a few days’ supply) will require more regular, evenly spread deliveries than a plant with a large storage capacity. Low levels of stockholding at the power station will increase the importance of reliable and flexible transport. Reducing the environmental impacts of biomass transport and storage Transport and storage components of the supply chain for biomass fuels can be responsible for a significant proportion of the total energy use and environmental impacts that arise in the biomass supply chain, including traffic generation, vehicle emissions, vehicle noise, visual intrusion, water pollution and the health and safety of workers and the public. Costs of biomass fuel supply #p#分頁(yè)標(biāo)題#e#
IJPDLM 28,6 While other activities in the supply chain also result in environmental impacts (for example, fuel use, emissions and noise from farm and forest harvesting equipment) there is relatively little scope to reorganise these activities in such a way as to reduce their environmental effects. However, in the case of transport and storage activities there are a number of steps and decisions that can be taken in planning and operating the supply chain to reduce environmental impacts. These include factors such as: . vehicle selection; . vehicle routeing and scheduling; . storage and depot location; . landscaping; . load protection and security. The lorry movements required to move biomass from farms and forests to the power stations are likely to be more visible to local residents and to impose environmental impacts on a wider group of locals than any other activities in the biomass supply chain. The manner in which transport activities are conducted will have an important impact on the public’s perception of the supply chain. Public perception often proves to be a significant factor in the acceptability and future development of an industrial or commercial activity and can influence locational choices and land-use and transport planning decisions. Transport activities should therefore be conducted as efficiently as possible in order to minimise their environmental impacts (this may well have the added incentive of producing lower transport costs). In contrasting the environmental impacts of transport activity in biomass fuel with that of fossil fuels, it should be recognised that the road-based biomass supply chains have to be set against the rail or pipeline-based systems which are often available for coal and oil/gas supplies, respectively, and which in themselves are more fuel efficient and less environmentally damaging.
Modelling the delivered costs of biomass supply chains 生物質(zhì)供應(yīng)鏈的交付成本建模
In order to explore the total delivered costs of biomass fuel associated with different supply systems and to be able to make cost comparisons between systems for one fuel and between different biomass fuel types, it was necessary to develop what we have referred to as “supply chain option models”. These models assist in building up an overall understanding of the logistics costs of supplying fuel from a farm or forest to a power station through a detailed analysis of where costs arise in the supply system and the relative importance of different cost components and activities. The supply chain option models can be used to obtain an understanding of the delivered cost of different supply chain options for each biomass fuel and to compare the delivered costs produced by different supply systems for each fuel. The models also make it possible to compare supply chain options and the associated costs for different biomass fuels. The supply chain option models take account of the all activities #p#分頁(yè)標(biāo)題#e#
from harvesting through to delivery at the power station and the unloading of the transport vehicle. The modelling was undertaken using a spreadsheet package; these tools are extremely useful for decision support systems, scenario modelling and sensitivity analysis (Coles and Rowley, 1996). Cost calculations used in the model are based on the cost of supplying one tonne of dry matter to a power station[2]. This cost is based on the time that any necessary supply activity takes to complete (and from this it is possible to calculate the cost of that activity given the hourly operating cost of equipment used and the cost of labour required) together with other input costs that go to make up the delivered cost (e.g. the cost of purchasing/growing biomass fuel). It is then possible to derive the cost per tonne of dry matter for that particular activity. The delivered costs produced by the models refer to the costs of supplying the fuel on a dry matter basis; they do not therefore include profit margins for contractors (forestry, agricultural and transport contractors). The supply systems modelled for each fuel were intended to represent efficient and cost-effective ways of supplying biomass fuel to power stations in schemes in which the fuel is used to generate electricity. Power stations will require year-round supply of large quantities of biomass on a regular and timely basis. Rather than being supplied to the power station from a market, the biomass will be supplied directly by contracted farmers, foresters and fuel suppliers. For these reasons, biomass fuel supply is best thought of as an industrial process rather than as a traditional agricultural or forestry operation. In order to be cost-effective these supply systems will need to exhibit several features. First, they will make use of capital-intensive, high productivity machinery. Second, efforts will be made to minimise the downtime of expensive equipment and hence maximise equipment productivity. Third, for the purposes of fuel supply they will make use of the expertise of specialist contractors (agricultural, forestry and transport) rather than farmers and small-scale forest owners (there are, however, opportunities for the latter to be involved in growing fuels and making fuel resources and/or land available). Fourth, the supply systems will be planned using an entire supply chain perspective from point of harvest through to delivery at the power station; the fuel supplier will therefore need to take account of all activities necessary to achieve fuel supply and integrate the necessary supply chain activities in a smooth and co-ordinated manner. It is important to note that the supply chains modelled for biomass fuels do not currently exist. Therefore in order to conduct this work and collect suitable data it was necessary to draw on data from existing operations carried out for other purposes (e.g. straw supplied to other markets), published data from trials (e.g. forestry and coppice harvesting trials), and to carry out interviews and discussions with people with recognised expertise with respect to each of the different biomass fuels or the activities or equipment associated with their supply. In addition, the project team had to use their own expertise and best judgement to determine suitable parameters[3]. Costs of biomass fuel supply #p#分頁(yè)標(biāo)題#e#
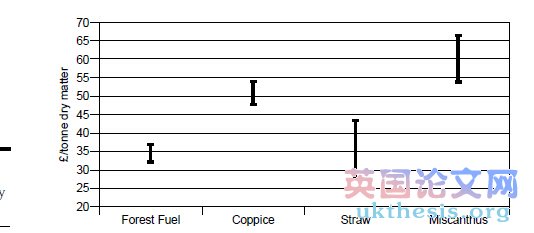
IJPDLM 28,6
Results of the supply chain option modelling 供應(yīng)鏈期權(quán)模型的結(jié)果
The results from the modelling of delivered costs for a range of supply systems for forest fuel, short rotation coppice, straw and miscanthus are summarised below and the comparison of delivered costs calculated for all of the fuels is discussed. Forest fuel Five systems for supplying forest fuel from the forest to a power station were modelled. It was assumed that, except in the case of young trees extracted to help the remaining trees grow, only the brash from the trees is used for energy production; the stem of a mature tree is used in higher value wood industries. Four of the systems modelled involve either cutting down the whole tree or just the brash and then extracting this to the forest landing (a cleared area in the forest that can be accessed by road vehicles). In the case of whole tree harvesting, the brash has to be separated from the stem at the landing. The forest fuel can then either be chipped at the landing and transported to the power station, or transported unchipped (and then processed at the power station using a centralised chipper – it has to be chipped before it can be used at the power station). The use of intermediate storage, in addition to storage at the forest landing, was also modelled. The fifth supply system examined involves chipping the forest fuel at the time of harvesting. In this system the material is chipped at the tree stump by a forward-mounted chipper and transported directly to demountable containers at the roadside which are collected by a road transport vehicle and then stored at an intermediate point before being transported by road to the power station. The difference between the costs of the forest fuel supply systems modelled were less than between the supply systems for the other biomass fuels considered in the study (ranging from £32 to £37 per dry tonne for forest fuel). The fifth supply system produced the highest delivered cost of all the systems considered. This is due to the two road transport stages and doubling handling of the wood chips that is necessary when intermediate storage is used. Short rotation coppice Five supply systems were modelled for the supply of short rotation coppice supply from the field to the power station. Two involve direct cut and chip harvesting (one using on-farm storage of the chips and the other intermediate storage before delivery to the power station). The other three systems examined are stick harvesting systems in which the sticks (four to five metre stems of willow and poplar) are stored on-farm until required for delivery to the station. The sticks would then either be chipped and transported or transported as whole sticks. The difference in delivered cost between the cheapest and most expensive supply systems modelled for coppice was not very large. Delivered costs in the cheapest system were only 13 per cent lower than the most expensive system. The results indicate that the delivered cost of fuel produced using a direct cut and chip harvesting supply systems and stick harvesting supply systems are #p#分頁(yè)標(biāo)題#e#
broadly similar. However, if decomposition of chips during storage can be Costs of reduced then direct cut and chip systems would be able to achieve lower biomass fuel delivered costs than stick harvesting systems. supply Straw Five supply systems were modelled for straw supply. As well as supply systems for large Hesston rectangular bales which are commonly used in biomass fuel 1.2 metres ′ 1.2 metres supply systems in Denmark (measuring 2.4 metres × and weighing approximately 500 kilograms each), supply systems for small rectangular bales and roll bales were also modelled. The results of the modelling show that straw supply systems producing large Hesston bales have substantially lower delivered costs than systems involving the production of small rectangular bales or roll bales. The modelling suggests that intermediate storage of large Hesston bales (i.e. two road transport movements and double handling) will have delivered costs that are approximately 10 per cent higher than systems involving on-farm storage and then direct road transport to the power station. Miscanthus Miscanthus, a perennial tall grass, has received a significant degree of attention in relation to its use in biomass fuel systems due to its high theoretical crop yield potential. However, there has been very little research into the potential supply systems for miscanthus if it was used to produce electricity at power stations. Therefore, in developing strategies for miscanthus it was necessary to devise supply systems that it was felt could be established. Two miscanthus supply systems were modelled. One involves direct cutting and chopping of the miscanthus (similar to that for short rotation coppice) using a modified forage harvester. After storage on the farm the chopped material would be transported to the power station. The second system involves producing bales from miscanthus. The miscanthus would be mechanically cut and left in the field. Baling machines as used in straw bale production, would then be used to produce large rectangular Hesston-sized miscanthus bales. After stacking and storage, the bales would be transported to the power station. The modelling indicates that a supply system producing baled miscanthus is likely to have lower delivered costs than a direct cut and chop system. In the modelling, the delivered costs per tonne of dry matter are approximately 20 per cent lower for baled miscanthus than for chopped material. Comparison of delivered costs of biomass fuels The results of supply chain option modelling suggest that the total delivered costs and the breakdown of these costs between activities are, as would be expected, significantly different from one biomass fuel to another. The range of delivered costs produced by the supply systems modelled for each biomass fuel are shown in Figure 2.
IJPDLM 28,6 Figure 2. The range of delivered cost for biomass supply systems £/tonne dry matter 70 65 60 55 50 45 40 35 30 25 20 Forest Fuel Coppice Straw Miscanthus The modelling work indicates that the delivered cost per tonne of dry matter for large rectangular Hesston bale straw systems are lower than the costs of other biomass fuel supply systems. This is due to two key factors: first, straw is a waste by-product and its growing costs are allocated to the cost of the crop produced, and second, that such straw supply systems already exist commercially, serving markets such as the animal feed and bedding and the mushroom composting industry. Therefore these supply systems have been subject to a long period of supply chain planning and machinery developments in order to make them as efficient as possible. The same is not true (or at least not to the same degree) of the supply chains for other biomass fuels. Forest fuel supply systems produced the next lowest delivered costs per tonne of dry matter at the power station in the modelling. Centralised chipping of forest residues at the power station has operational advantages in comparison with chipping in the forest that could make it an attractive forest fuel supply system to the power station operator. It gives the power station operator far greater control over the chipping process and hence the size distribution of the chips. The size and quality of the chips that are fed into the boiler may, depending on the conversion technology used, be critical to generation efficiency and the smooth running of the power station. The delivered costs for the short rotation coppice supply systems modelled were, on average, approximately 50 per cent greater than the delivered costs of forest fuel supply systems. This cost difference is due to a number of factors, but the main difference between these two fuels is that coppice has to be grown specifically for biomass supply and therefore the costs of this are all attributable to the biomass supply system (this is also true of miscanthus), whereas forestry material for energy production is a waste by-product and the costs of growing this material are not attributable to the biomass industry. For all biomass fuels in which the use of intermediate storage systems have been modelled, this supply system, in which the fuel will have to be transported twice by road transport vehicle (first from farm/forest to intermediate store and then after storage from store to power station), will result in a higher delivered cost than a system in which there is only one road transport movement (direct #p#分頁(yè)標(biāo)題#e#
from farm/forest store to power station). Use of an intermediate store will add in the region of 10 per cent to 20 per cent to delivered costs, as a result of the additional transport and handling costs incurred.
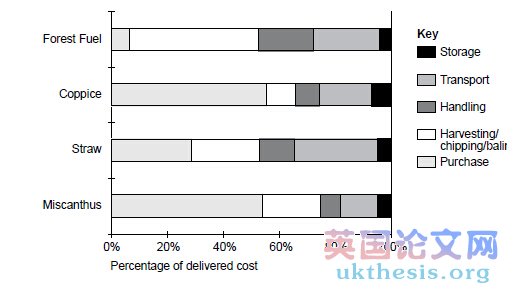
Figure 3 illustrates the proportion of total delivered cost accounted for by each of the activity cost components for the cheapest supply system modelled for each biomass fuel, assuming that the fuel is transported 40 kilometres to the power station. It can be seen that the importance of these cost components vary substantially from one biomass fuel to another; significant variations in the relative importance of cost components also exist between one supply system and another for each fuel.
Conclusion 總結(jié)
By taking an entire supply chain perspective of biomass supply from point of harvest through to delivery at the power station, as in this work, it is possible to consider the interrelationship between all the activities necessary in order to deliver fuel in an efficient manner and the delivered costs of different supply systems. The work has shown that logistics costs represent a significant proportion of total delivered cost in biomass supply. The cost of transporting and handling biomass fuel from its point of availability (in the field in the case of straw, miscanthus and coppice and in the forest for forest fuel) to its point of utilisation at a power station are activity cost categories that are of great importance in all biomass fuel supply systems. These two activity costs are likely to represent about 50 per cent or more of delivered straw costs, approximately 50 per cent of delivered cost in forest fuel supply, 35 per cent or more of delivered costs for coppice and 20 per cent to 40 per cent of delivered cost in miscanthus supply systems. While the transport costs of supplying biomass fuels to power stations are related to the number of lorry movements required, other important factors also affect transport costs (such as the distance over which the fuel has to be moved KeyForest Fuel Storage Transport Coppice Handling Harvesting/ Straw chipping/balin Purchase Miscanthus 0% 20% 40% 60% 80% 100% Percentage of delivered cost Costs of biomass fuel supply Figure 3. The proportions of delivered cost accounted for by each activity for the lowest cost supply system for each biomass fuel
IJPDLM 28,6 and the terminal time – i.e. the time spent loading and unloading vehicles). Biomass fuels with relatively high bulk densities (such as coppice and forestry residue chips) are likely to require fewer lorry movements to deliver a specified tonnage to a power station than biomass with lower bulk densities (such as miscanthus and straw). The local traffic impacts generated by this transport activity will vary from one biomass scheme to another and will depend on the abundance of biomass fuel in the area and the existing road infrastructure. However, as shown in Table I, the number of daily transport deliveries to the power station are unlikely to be greater than for many other industrial activities. In short rotation coppice and miscanthus supply systems the major cost component is likely to be the purchase/growing cost of the fuel (this can account for up to approximately 50 per cent of delivered cost per dry tonne). There may be relatively little scope to alter this cost and it is therefore essential that attention is focused on the efficiency of activities such as transport and handling (together with harvesting technology and operation) if delivered costs are to be reduced in the future. Mature supply systems such as those already operated in straw supplied for mushroom composting and animal feed and bedding have proved that organising and managing the supply chain efficiently can result in relatively low delivered costs. In these straw supply systems a major straw supplier either personally carries out, or works closely with contractors to undertake, all the activities from baling through to delivery to final customer on several hundred farms within a straw-producing region of the UK. This can involve the supply of tens of thousands of tonnes of straw per year and, given this throughput, allows the contractor to utilise high productivity specialist machinery. Therefore efforts should be placed on trying to achieve similar efficiency in transport and logistics activities in other less established biomass supply chains. In devising a supply strategy for a power station, it is likely to be necessary to operate a range of different systems in order to ensure that biomass supply can be maintained all year round. For example, a fuel supplier supplying straw will probably have to make use of an intermediate store supply system as well as a farm store supply system. Although according to the result of the modelling the farm store system would be preferable to intermediate storage in terms of delivered cost, it is unlikely that farm stores could be accessed by road transport vehicles during wet winter periods and therefore intermediate stores would also have to be available. The management of biomass fuel supply chains will have to be organised in a co-ordinated and thoroughly planned manner if the fuel is to be delivered to the power station at the times required each day and at the correct fuel specification on a year-round basis. Rather than each activity in the chain being planned and conducted in isolation, it is extremely important that the supply chain (from point of production through to supply to a power station) is viewed as a whole. Only in this way will it be possible to achieve integration between activities and hence efficiency in the supply of biomass fuels. Given the number of different parties involved in the supply of fuel, and their different perspectives and scale, the supply chain management will be of the utmost importance. #p#分頁(yè)標(biāo)題#e#
All parties need a detailed knowledge of upstream and downstream activities in the supply chain when determining how best to conduct their own work and responsibilities. Partnerships and working alliances need to be established and encouraged as supply chain efficiency in both economic and environmental terms requires the active involvement and efforts of all parties. Notes 1. It is beyond the scope of this paper to discuss in detail the supply chain modelling of each of the biomass fuels included in the project. The full report, listed in the references (Allen et al., 1996), and available from ETSU, contains this information. 2. The cost of supplying fuel to the power station, calculated per tonne of dry matter. This is not to suggest that oven dry biomass is supplied to the power station; wet biomass is supplied but our cost calculations are based on the dry matter present in the biomass. 3. A number of biomass fuel-specific assumptions were made with respect to the characteristics of each biomass fuel, the equipment and facilities used in its supply and the operating costs and productivity rates. These assumptions are too numerous to list here and anyone interested in these should refer to Allen et al. (1996), in which they are fully documented. However, a number of important assumptions were made in the modelling that were common to all biomass fuel supply systems, including: (i) the road transport distance from the farm or forest to the power station was set at 40 kilometres; (ii) the delivered costs are based on round trip distances as the lorries will be travelling from farms/forests to the power station and back again in biomass supply systems (i.e. 80 kilometres); (iii) wherever possible use is made of the largest road transport vehicles possible (in terms of volume and/or gross vehicle weight) in order to minimise the unit costs of road transport; (iv) the fuel storage period has been set as six months, as the biomass will generally be harvested at a specific time of year but will have to be supplied to power stations in constant quantities on a regular, year-round basis; (v) biomass will be stored in the open air (i.e. no use will be made of covered buildings and/or ventilated systems) due to the high cost of these options; (vii) in chip storage systems dry matter losses are assumed to occur at a constant rate of 4 per cent per month and in bale storage systems the entire top layer is assumed to be unsuitable for supply; (viii) the delivered cost calculations do not include a profit element for contractors or the fuel supplier.
References 文獻(xiàn)
Allen, J., Browne, M., Hunter, A., Boyd, J. and Palmer, H. (1996), Transport and Supply Logistics of Biomass Fuels: Volume 1 – Supply Chain Options for Biomass Fuels, (Report No. ETSU/B/W2/00399/REP/1), ETSU, Harwell. Ballou, R. (1992), Business Logistics Management, Prentice Hall, Englewood Cliffs, NJ. Christopher, M. (1987), Effective Logistics Management, Gower, Aldershot. Coles, S. and Rowley, J. (1996), “Spreadsheet modelling for management decision making”, Industrial Management and Data Systems, Vol. 96 No. 7, pp. 17-23. Cooper, J. (Ed.) (1993), Strategy Planning in Logistics and Transportation, Kogan Page, London. Department of Trade and Industry (1994), New and Renewable Energy: Future Prospects in the UK, Energy Paper Number 62, HMSO, London. ETSU (1994), Energy Technologies for the UK: An Appraisal of UK Energy RDD&D, ETSU, Harwell. United Nations (1991), Green Energy: Biomass Fuels and the Environment, United Nations, Geneva. Costs of biomass fuel supply #p#分頁(yè)標(biāo)題#e#